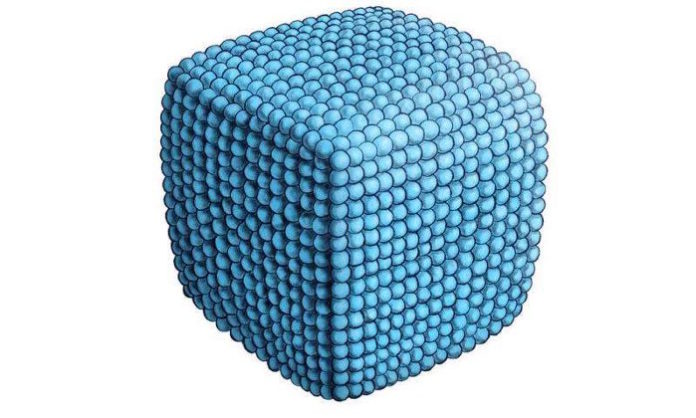
Phospholipids play an important role in living organisms, forming membranes, among other things. The new findings enhance the understanding of the forces acting within biological membranes and could open up new pathways in medicine. The researchers headed by Andreas Zumbühl from the University of Fribourg in Switzerland present their results in the journal Angewandte Chemie.
Their special chemical structure allows phospholipids to self-assemble to form membranes consisting of two connected layers of molecules. These are a key component of the biological membranes that separate the various parts of a living cell. Membranes made of phospholipids can also automatically form three-dimensional, enclosed structures, for example in water where they produce so-called vesicles.
Normally, such vesicles are spherical in shape, to minimise the surface tension. However, the 1,2-diamidophospholipid now analysed by the scientists produces cuboid vesicles at room temperature. This is because this phospholipid forms very close-packed and therefore very stiff layers, which are very difficult to bend, thanks to special bonds, known as hydrogen bonds, which minimise the distance between the molecules. When it assembles as a three-dimensional structure, the membrane continues to favour flat surfaces and structures having as few edges as possible, conditions that are satisfied by a cube.
Its unusual structure could make this phospholipid interesting for medical applications, for example to deliver drugs to specific parts of the body. “The edges of the cube are formed by the outer molecular layer, whereas the inner layer has a discontinuity here. This membrane defect means that the structure may break there if the cube is shaken,” explains Zumbühl. A drug that has been encapsulated in the cube can therefore be released in a controlled fashion. “One might for example encase a drug that dissolves blood clots and use this in an emergency after a heart attack. High shear stresses would be exerted on the cube in a blocked artery, releasing the drug at precisely the location where it can do the most good,” says Zumbühl. The cube currently being studied is not itself suitable for such applications, however, since it is as yet too fragile.
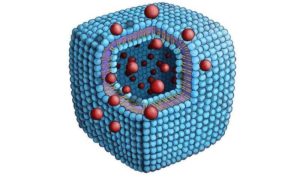
For the team of research scientists, the phospholipid examined is most of all an important step on the way to a greater objective: “We would like to understand what forces are acting in the membrane, so that we can later deliberately influence these. This would allow us to use phospholipids as a kind of building material, so as to construct specific structures on a cellular level,” says Zumbühl. To understand the precise details of the phospholipids, the scientists synthesise certain molecules, modifying their structure and properties slightly each time, in order to see what effect this has. Because a small change in the structure of a phospholipid can have a large effect.
The beamline P08 at DESY’s X-ray source PETRA III had to be specially equipped for these kind of structural investigations at the boundary between air and water. “Thanks to the optimisation of our set-up and the exact control of the temperatures and pressures acting on the membranes, even the surface pressure in an individual layer of the 1,2-diamidophospholipid could be determined,” explains beamline scientist Olof Gutowski from DESY, who made these measurements possible. The result surprised the scientists: “For 30 years, it has generally been assumed that the pressure in a biological membrane must be relatively high, around 30 Millinewton per metre,” says Zumbühl. “In the membrane we studied, however, the pressure must be considerably lower, around 5 to 10 Millinewton per metre. This calls into question the long-standing rule of thumb.”
Source: Phys.org